Dive into DSP in the final edition of our Demystifying SynBio series. If you’re just joining us, be sure to catch up on parts I, II, III, and IV.
Upstream bioprocessing – better known in biomanufacturing as fermentation – is directly followed by downstream bioprocessing (DSP). Just as fermentation is all about maximizing the production of a target compound, DSP focuses on capturing and purifying as much as possible, as cost effectively as possible. Distillation is a well-known example of downstream processing whereby alcohol from industrial fermentation is concentrated and purified to make whisk(e)y, gin, and other beverages. The role of DSP in synthetic biology product manufacturing is critical for commercial viability as it ensures the product meets quality and market standards, setting it up for long term success. Thus, developing a DSP strategy is often the first port of call when embarking on a biomanufacturing venture to ensure the technology and infrastructure exists to capture and purify the compound of interest.
In this fifth and final installment of our Demystifying Synthetic Biology series, we explain why DSP should be introduced as early as possible in the product development process, and we look at the range of processing techniques for large-scale fermentations. We also share the considerations and concerns in processing synthetic biology products, how synthetic biology and chemistry can work together for difficult-to-engineer compounds, and insights on integrating synthetic biology-enabled compounds into commercial product pathways.
The critical role of downstream processing
The ability to separate and process compounds to specific yields and purity levels can make or break the commercial viability of a synthetic biology product. The DSP phase focuses on separating and purifying a compound of interest, often from a complex fermentation broth. This process must ensure that the level of purification is both a high enough yield for commercial viability and within specifications for its final application, whether that is formulation into a food, therapeutic, or substance for another manufacturing process.
A successful downstream process ideally starts before product development has even begun. Knowing the physical and chemical properties of a target compound and the anticipated fermentation methods allows the DSP team to predict how it can most effectively be purified and – critically – how much it will cost. Understanding this cost is important for determining the viability of a product and process.
Modeling and small scale data is crucial to estimating these costs. It is far too expensive to test and troubleshoot fermentation and DSP methods at a manufacturing scale of thousands of liters. Instead, we’ve developed methods to test at the benchtop scale and capture data on the compound and the fermentation broth. An expensive DSP cycle, or one that results in poor yields or quality, will ultimately result in a commercially non-viable product and wasted resources and time.
The philosophy of “Begin with the end process in mind” lies behind every successful DSP. Constant communication between the DSP team, the upstream fermentation team, and the strain engineering team is critical in implementing this philosophy and bringing a product to market. For example, the DSP team must know if any additional solvents have been added to the fermentation broth in the development phase – solvents such as DMSO may help fermentation broth ingredients dissolve in the culture media, but can also make product purification more difficult for the DSP team.
Once the downstream process is defined and demonstrated to work, it is deployed at the manufacturing scale. Refinements to the process are required frequently for cost-cutting and for adapting to changes in the fermentation process, such as different media components or even newly improved strains of the engineered organism. These changes are conducted at the benchtop scale prior to implementation in manufacturing.
Product is key in DSP
The chosen method of DSP depends largely on the target compound and how it is produced. During upstream fermentation, engineered organisms typically excrete the product extracellularly into the fermentation broth, which is composed of a complex mixture of off-target metabolites and media components.
The DSP strategy required to extract and purify the product is driven by the fundamental physical properties of the target compound itself. The separation process for extracellular products begins with separating the solid biomass from the liquid fermentation broth. Centrifugation separates the dense biomass from the culture supernatant containing the product – in other words, separates the solids from the liquids. This remains quite dilute in terms of volume-to-product, so the following step involves reducing the volume. This is where three fundamental properties of the product – size, solubility, and polarity – come into play.
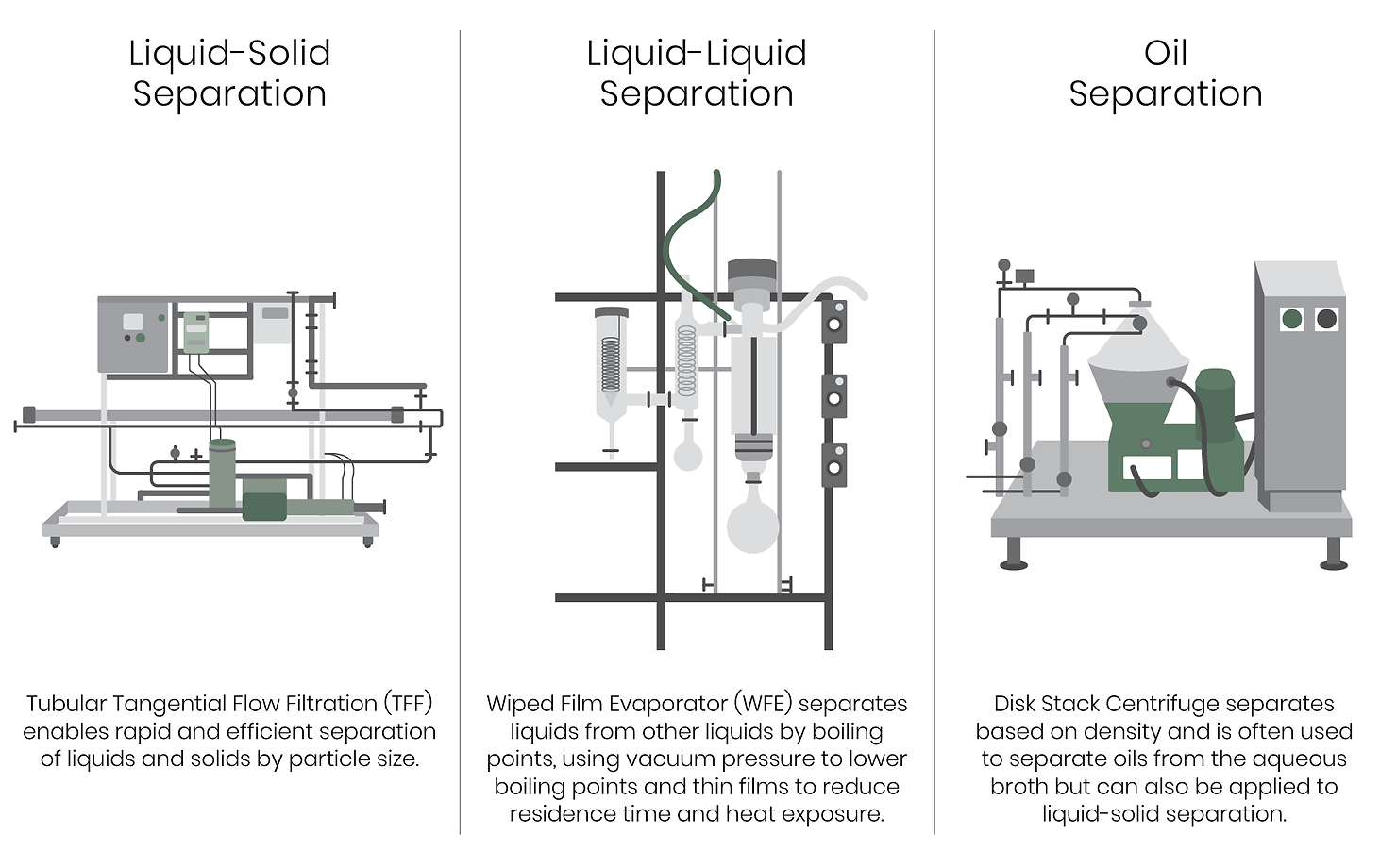
Considerations for DSP of synbio products
Synthetic biology offers a fast, cost-effective route to a wide array of chemical and biological compounds. Like all technologies, synthetic biology has its own unique challenges and limitations that must be accounted for in DSP:
1. Compounds may not be fully excreted by the cell
In cases where the organism retains the target compound intracellularly, cell lysis – the breaking down or disruption of the cell membrane chemically or mechanically – may be necessary for the DSP. Cell lysis often complicates the process by adding more contaminants to the mix, making purification more difficult. Fortunately, synthetic biology allows for improved strain iterations, and researchers can also engineer solutions to improve product excretion and overall titer in the fermentation broth.
2. Compounds may be toxic to the production organism
In some cases, the target product may be toxic to the organism producing it. Bioprocessing solutions, such as overlaying the fermentation culture with an oil that absorbs the target compound, can mitigate the toxic effects but may significantly add to the cost of DSP depending on the methods required. Synthetic biologists may work around this by engineering an organism with an additional chemical group that blocks the toxic group on the target compound. An enzymatic or synthetic chemistry step can alter this group and convert the fermentation product into the desired final product during the downstream process.
3. Finding a clear, integrated to-customer route
A longstanding hurdle for synthetic biology products is integrating them into existing process engineering infrastructures. In the late 1990s and early 2000s, the algae biofuel boom fell apart when the complex downstream processing steps required to make these products usable for cars became so costly at scale – coinciding with a drop in oil prices at the time – that these products became economically non-viable. Ensuring that fermentation and downstream processes fit existing infrastructures helps clear a route to customer application, making synthetic biology-designed products commercially achievable and feasible. This is exemplified by Amyris’ squalane and Genomatica’s nylon building block, hexamethylenediamine, both produced via fermentation.
Synthetic biology demystified
Synthetic biology – building with the power of biology – is not a new idea. The misconception and myth that the technology cannot scale are perhaps the most commonly misunderstood things about synthetic biology. Throughout this blog series, we have demonstrated that synthetic biology is like any other field of science – built on the shoulders of giants – in what is simply the next iteration of the field of biology. The field combines a rational, engineering approach to research and product development with refinements on decades-old molecular biology tools (as well as more recent ones). These tools are further enabled by modern technologies like automation, software, and computational biology to rapidly scale experimental design and execution in R&D.
Successful synthetic biology products on the market today rely on integrating into the existing infrastructure of upstream fermentation bioprocesses and downstream purification processes. These products – present in industries as diverse as fuel, food, pharmaceuticals, cosmetics, textiles, and more – are no longer a whispered myth among researchers or a hyped-up presentation at industry conferences.
Synthetic biology is here now. The field is advancing steadily, delivering on its potential to develop effective, sustainable, and economical bio-based alternatives for essential chemical and biological compounds.
Follow Antheia on LinkedIn to stay up to date on our newest blog content and job openings.